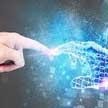
Virtual reality (VR) and augmented reality (AR) are some of the hottest multidisciplinary technology trends right now, integrating computer, various sensor, graphic image, communication, measurement and control multimedia, artificial intelligence, and other technologies.
VR commonly employs behavioral interfaces and computer programs to allow users interact with each other and to simulate the behavior of 3D objects in a virtual environment. VR implies a complete immersion experience that shuts out the physical world.
In contrast, AR adds digital elements to a live view often by using the camera on a smartphone. Examples of augmented reality experiences include Snapchat lenses, the game Pokemon Go and navigation systems that superimpose a route over the live view of the road.
While AR can work on existing display screens such as smartphones and other monitors, most people associate VR with more or less bulky headset displays.What that means is that mimicking human sensory systems at the moment is often limited to audio and video channels, and requires boxy and rigid devices.
Interactive textile electronic devices will provide suitable platforms for VR/AR applications because of their excellent performance and unique immersive features such as lightweight, handiness, flexibility, comfort, and low strain even under high deformations.
Textile-based systems offer a better experience in emerging VR/AR applications with respect to other sensory interactions than just audial and visual interactions. Furthermore, textile devices enable the wearable VR/AR system to be smaller, softer, and more comfortable, which broadens the range of VR/AR applications.
Intrinsic conductive nanomaterials such as carbon nanotubes and metallic nanowires as well as nanoscale devices such as triboelectric nanogenerators are key to developing these electronic textiles.
A review article in Advanced Functional Materials (“Textile Electronics for VR/AR Applications”), compiled by researchers from The Hong Kong Polytechnic University, address the state-of-the-art textile-based VR/AR devices, systems, and applications.
The authors first introduce the field of textile electronics (e-textiles) including their definition, classification, fabrication technology, and system architecture.To catch up on the latest developments in e-textiles and wearable electronics) take a look at our collection of Nanowerk Spotlights on the subject.
Apart from actuators and electrodes, an important part of e-textiles are sensors to detect physical and chemical stimuli to acquire abundant information about the environment as a basis of interaction. Considering the characteristics of textiles, a sensor in textile format can be flexible, durable, biocompatible, and lightweight. The sensors can be used in VR/AR systems as strain, pressure, temperature, gas, smell, and multifunctional sensors.
For instance, strain sensors can transduce mechanical motion into an electrical signal to monitor large-scale and small-scale motions; these may find applications in healthcare, sports, and robotic industries.
Sensory stimuli provide people with a coherent perception of the world. Textile electronic devices are an excellent platform for people to simultaneously experience audio, video, allowing haptic, multimodal sensory inputs, and movement in graphically rendered objects or environments. Perception devices transform various perception models in the virtual world to multi-channel stimuli (e.g., tactile, visual, and hearing signals) for detection.Following their discussion of e-textiles, the authors then introduce interactive textile devices used in VR/AR systems. This is followed by a presentation of various fabrication processes and examine certain VR/AR systems integrated with interactive textile devices, electronic interfaces, power supplies, and display hardware.
To fabricate different textile electronics with advanced electrical and textile properties, researchers have explored different fabrication methods. The techniques can be generally categorized into two groups: physical methods and chemical methods.
The former includes spinning, printing, coating, and physical vapor deposition. The printing method has the advantages of low temperature, non-vacuum operation, low production cost, potential for large-scale manufacturing, and wide compatibility with materials.
Chemical techniques involve chemical reactions to modify the textile surfaces of conductive materials by adding chemicals or controlling the chemical components of a substrate. The common chemical fabrication methods include plating, chemical vapor deposition (CVD), and carbonization.
One of the key components – and also a significant challenge for making devices truly wearable – is the energy supply for all the components of VR and AR systems – display units, controllers, analog-to-digital converters, wireless modules, sensing units, and computing units.
Each of these components can work separately or with other components through wireless/wired communication technologies. They also can be powered separately as they need different levels of power consumption. Consequently, flexible batteries and supercapacitors are suitable for higher power consuming devices such as displays, while fabric-based triboelectric generators are promising systems for application in self-powered sensing and energy harvesting.
For instance, last year researchers demonstrated an all-textile triboelectric sensor-based smart glove that was capable of capturing human gestures for remotely controlling a VR that enabled a teleoperated robotic gripper (Journal of Materials Chemistry A, “An all-textile triboelectric sensor for wearable teleoperated human–machine interaction”).Although VR/AR systems cannot be completely fabricated in textile structures (yet), the authors believe that adopting certain developed textile electronic devices can provide further user-friendly immersive VR/AR experiences.
Concluding their review, they note that the major hindrances today to the progress of textile electronic VR/AR systems are insufficient performance, immature production technology, and lack of system-level investigations and standards. Also, the general lifetime of textile electronics is shorter than that of rigid microelectronic devices and many textile electronics produced from organic or polymer functional materials are sensitive to water and oxygen, and the laundering process of textiles can cause deterioration of function.
As is typical for this kind of early stage technology, most textile electronics experiments have been conducted in the laboratory or research stage, and very few textile electronics have been commercialized so far because many devices were made using laboratory-type manual techniques instead of factory-type machinery.
So here we are, at the end of 2020, and the answer to that same question still is inconclusive.
The promise of nanomedicine is quite broad – ranging from improved, less toxic, more targeted and even personalized medicines, to more sensitive and cheaper diagnostic tools, innovative structural materials and the prospect of cellular and tissue repair systems.