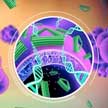
So here we are, at the end of 2020, and the answer to that same question still is inconclusive.
The promise of nanomedicine is quite broad – ranging from improved, less toxic, more targeted and even personalized medicines, to more sensitive and cheaper diagnostic tools, innovative structural materials and the prospect of cellular and tissue repair systems.
To date, however, most nanomedicines have been focused on cancer research. Although thousands of anticancer nanomedicines have achieved outstanding success in preclinical cancer models, few anticancer nanomedicines have been approved by regulatory agencies.
This low success rate has provoked decades of debate regarding the effectiveness of anticancer nanomedicines. A comprehensive analysis of anticancer nanomedicines (Nature Reviews Materials, “Analysis of nanoparticle delivery to tumours”) found low tumor delivery efficiency (<0.7%), leading to extensive discussion regarding the delivery effectiveness of anticancer nanomedicines.
The striking imbalance between the ever-increasing number of preclinical studies reporting the development of ever more complex nanomedicines on the one hand, and the relatively small number of nanomedicine products approved for clinical use on the other hand, has become the focus of intense debate (see for instance: Expert Opinion on Drug Delivery, "Cancer nanomedicines: oversold or underappreciated?"; Nature Reviews Materials,"Evaluation of nanomedicines: stick to the basics" and "Reply to 'Evaluation of nanomedicines: stick to the basics'").
And it's not that the field hasn't received significant funding. In the U.S., for instance, the National Cancer Institute's Centers of Cancer Nanotechnology Excellence (CCNE) received $165 million funding during their 15-year lifetime (2005-2019). This enabled the CCNEs to obtain more than $500 million of other federal grants along with more than $1.48 billion in private funding. So that's well over $2 billion of funding in the U.S. alone.
A recent Perspective in ACS Nano ("What Went Wrong with Anticancer Nanomedicine Design and How to Make It Right") takes a look at current design criteria of anticancer nanomedicines and debates their validity in a clinical context.
Most anticancer nanomedicines follow three basic criteria with the intent to improve anticancer efficacy and to reduce toxicity:
1) Nanomedicines increase drug accumulation through enhanced permeability and retention (EPR) in tumors to improve anticancer efficacy.
2) Long systemic circulation of nanomedicines with high plasma concentration reduces reticuloendothelial system (RES) clearance and decreases drug accumulation in the normal organs to reduce toxicity, and to enhance the EPR effect.
3) A universal nanodelivery platform based on EPR and long systemic circulation can be developed to deliver different anticancer drugs.
The authors of the ACS Nano article argue that "a universal nanodelivery platform that uses the same design criteria for different drugs is not feasible. Rather, drug-specific nanodelivery systems are required to overcome the intrinsic shortcomings of delivered drugs, which are determined by the physicochemical, pharmacokinetic, and pharmacodynamic properties of the delivered drugs and nanocarriers to improve their efficacy/safety."
Furthermore, citing a number of studies, they suggest that the debate over tumor EPR may have mixed two different questions and missed more clinically relevant comparisons for nanomedicines versus free drugs.
They also argue that long systemic circulation should not be a universal nanomedicine design criterion because it does not further improve tumor accumulation by tumor EPR in human patients nor universally reduce distribution in normal organs.
To address these issues, the researchers propose the creation of new nanomedicine design criteria:
Identify the intrinsic shortcomings of the delivered drugs based on their physicochemical, pharmacokinetic, pharmacodynamic, and unique efficacy/safety profiles.
Assessing the pharmacokinetic distribution of nanocarriers and how they alter the free drug disposition in the targeted organs to improve clinical efficacy/safety.
Studying the types of cells in the tumor microenvironment to which drugs will be delivered (e.g., cancer cells, endothelial cells, stromal cells, or immune cells) to improve drug efficacy.
"Three types of researchers, each with their own strengths and limitations, have been working on nanomedicine research in the past few decades," the authors observe. "Pharmaceutical scientists have strength in drug delivery and the clinical aspects of nanomedicine development; engineers have strength in engineering processes and the generation of novel nanostructures; and chemists (and material scientists) have expertise in generating new materials and new chemistries of the nanostructure. In general, these three fields have not worked together closely, and thus may not fully appreciate the others’ points of view."
In concluding their articles, they point out that neither defensiveness against critical evaluations of the current status quo nor simple dismissal of nanomedicine research is helpful. It will be important to critically evaluate outdated theories of applied nanomedicine research in order to avoid unnecessary and costly repetitions of failed ideas.
Since its first demonstration in 2004, the large-scale commercial production of graphene has proven difficult and costly (‘large scale’ usually defined as weights more than 200 mg or films larger than 200 cm2). For instance, at an estimated cost of $50 000 to $200 000 per ton for graphene powders and $45 000 to $100 000 per m2 of graphene film, industrial production methods and costs are restraining graphene utility.
Lire la suiteResearchers have created a unique device which will unlock the elusive terahertz wavelengths and make revolutionary new technologies possible.
Lire la suite